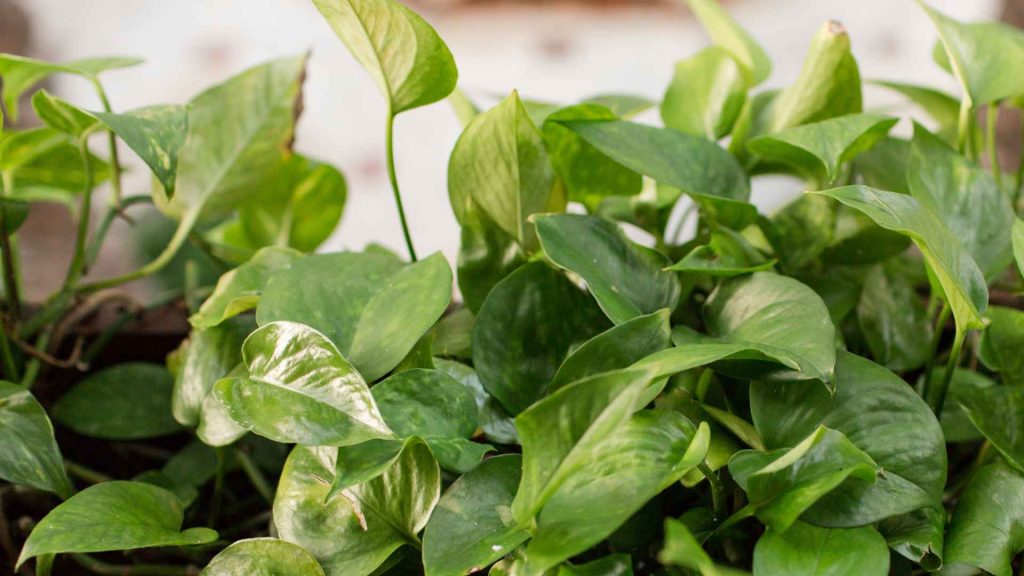
Plant phenotypes are quantitative characteristics such as growth rate, yield and stress tolerance that can be quantified at various scales of organization – from individual cells to entire canopies.
Modern phenotyping methods are non-destructive, high throughput tests that characterize plant traits with great accuracy and precision. They enable us to capture in just a few hours what used to take weeks or months of data collection in the past.
What is a plant phenotype?
Phenotypic data, or plant expression patterns, are the results of interactions between genotypes and environmental conditions. This knowledge can be valuable for crop improvement or plant breeders when selecting genetic variants that will thrive in various climates.
A phenotype is the outcome of a series of processes that sequentially alter a gene's composition (transcriptomics) and its protein counterpart (proteomics). These changes affect metabolites and ions in plants, leading to visible differences in their physiological state and morphology.
Phenotypic information about an organism is determined by multiple, dynamic feedback loops triggered by its environment. This interaction between genotype and environment produces a range of measurable characteristics we refer to as phenotypes, such as plant height, leaf count or biomass.
Phenotyping has traditionally been a time-consuming and laborious task due to the limited number of available data points. This has hindered matching phenotypic information with genomic data – essential for genome-wide association studies and plant breeding efforts. Thankfully, recent advances have enabled non-destructive image-based phenotyping approaches that enable high throughput analysis of plants. This technology helps identify genetic architectures controlling important traits, leading to faster breeding cycles for the acquisition of desirable traits.
How is plant phenotype measured?
Scientists have made major strides in identifying the genetic material of key crops, but are still lacking technology to reveal how those genes manifest themselves as observable traits in plants (phenotype). Phenotyping – measuring plant characteristics – can help scientists modify desirable traits, adapt climate adaptation strategies, increase crop yield and nutritional characteristics, as well as optimize management techniques for weed and pest control.
Traditional phenotyping requires manual data collection, which is time-consuming and laborious. Automated field phenotyping with sensors, configurable harvesting equipment, and drones produces far more data in a shorter amount of time than manual methods do. This enables researchers to bridge the genotype-phenotype gap faster which in turn leads to fewer breeding cycles for traits desired by researchers.
To maximize efficiency when connecting phenotypic information to genetic data, it is necessary to acquire and analyze multidimensional phenotyping data on different scales at various time points. This can be accomplished through integration of plant physiology, non-invasive phenotyping and functional genomics. This will create a robust approach for in silico assessment of plant responses under defined environments as well as crop modeling based on these insights.
What are some examples of plant phenotypes?
Phenotypes, also known as genotypes, are the physical and biological characteristics that result from the interaction between a plant's genetic background (genotype) and environmental conditions during development. Phenotypes vary between genotypes due to factors like temperature, light intensity, soil type and water availability.
Crop breeding utilizes plant phenotype to identify desirable genetic variations in target traits that can be selected to improve crop performance and boost profitability. Examples of different phenotypes in plants include drought tolerance, leaf water stress resistance, pathogen resistance, and increased grain yield.
Phenotypes are invaluable to precision agriculture and plant breeders, helping them select desirable varieties that can adapt well to specific environments at cost-effective costs. Although the field of plant phenotyping is still in its early stages, non-destructive image analysis-based phenotyping has already shown promise as a potential tool in this application.
Unfortunately, traditional phenotyping methods are time-consuming, laborious and expensive. Furthermore, they only cover a few phenotypes during key developmental stages of plants; thus resulting data that is often incomplete or uncertain hinders the acquisition of comprehensive phenotypic knowledge.